Why so few feral colonies?
Synopsis : With so many swarms lost by beekeepers, why are there relatively few feral colonies? Do they die from starvation, depredation or disease? What kills feral colonies?
Introduction
It can take a long time to understand complex natural phenomena. When you take into account geographic and seasonal variation you often end up with an explanation littered with more caveats than actual answers.
For example … do honey bees compete to the detriment of native solitary bees?
During May in an environment with limitless yellow acres of oil seed rape … probably not, but during early spring in an arable area with limited hedgerows … almost certainly.
Sometimes the explanation may seem obvious, but isn’t. Bumble bees restrict themselves to field margins whereas honey bees venture hundred of metres into the middle of a field of OSR. Not only are there huge amounts of pollen and nectar available, but the different species exploit it in different areas of the field.
Not simple and not necessarily obvious {{1}}.
Lost swarms
By many accounts, it’s been a very ‘swarmy’ season. The BBKA’s swarm line was swamped {{2}}. Many beekeepers ran out of equipment (and no doubt patience) hiving swarms lost by beelosers with poor swarm control.
With ~250,000 colonies in the UK I wouldn’t be surprised if there were 50-100,000 lost swarms and casts.
That being the case, why aren’t there more feral honey bees?
By feral I mean honey bees that were once managed and – through mismanagement? – are now no longer managed but are instead free living.
Why isn’t every suitable cavity – in church towers, hollow trees, outbuildings and roof spaces – occupied by ‘lost’ swarms?
Clearly there are some feral colonies, but they are sufficiently rare to be notable.
Those of you interested in feral colonies will also be aware of sites (cavities) that have been occupied by honey bees, but that currently are not.
The obvious explanation is that the pests and diseases – primarily Varroa destructor and the viruses that it transmits – that plague (and can destroy) our managed colonies have precisely the same devastating impact on feral colonies.
By definition, a feral colony is unmanaged. Therefore, any pests or diseases it carries when the swarm leaves the original hive should have an unrestricted opportunity to wreak havoc – and eventual destruction – on the newly established feral colony.
Pathogen loads and colony losses
The statement above is an assumption based upon a couple of observations:
- the majority of winter colony losses in managed colonies are due to Varroa transmitted deformed wing virus (DWV). This reduces the longevity of winter bees resulting in the colony shrinking to a size below that needed for viability, or – if it survives – incapable of building up in the spring (Dainat et al., 2012).
- limited studies of feral colony pathogen loads have shown higher levels of DWV than seen in managed colonies, but similar to the levels seen in unmanaged colonies (Thompson et al., 2014).
The relevant figure from the latter paper shows significantly higher DWV levels in feral (F) to managed (M) colonies.
In contrast, other pathogen levels tested – black queen cell virus (BQCV) and Nosema – were not significantly different.
The majority of well-read beekeepers are aware of the importance of managing Varroa levels. They also know what happens if the levels are not properly managed – or not managed at all.
Several years ago I presented graphs modelled using BBEHAVE software showing what happens over a 5 year period to colonies that are not treated to minimise Varroa levels.
I ‘primed’ these calculations with just 10 mites per colony … within two years mite levels exceed ~4000 and were four to five times that within three years (at which point total bee numbers start to fall).
By year four the colonies were managed by a mitekeeper, not a beekeeper, and colonies expired in the fourth or fifth year.
In reality, colonies die within four years because they usually start with many more than 10 mites … 🙁 .
Simples?
These observations, and those listed above, make it logical to assume that feral colonies do not litter the landscape because the mites and viruses kill ‘em.
In addition to pests and diseases we know that our managed colonies sometimes die for a variety of other reasons.
Queen failures, starvation, natural disasters (e.g. flooding or stampeding cattle) and stupidity {{3}}.
Other than stupidity I think it’s reasonable to assume that feral colonies probably experience a similar range of ‘threats’, though the stampeding cattle are unlikely to be a problem if they’re in the church tower.
OK?
Well, sort of. A lot of the above are assumptions. They’re based upon good observations and a reasonable understanding of the threats to managed honey bees, extrapolated to an unmanaged situation.
To be sure why there are fewer feral colonies than there are swarms lost we really need to observe some feral colonies, record which survive to the following season and look at differences between those that survive and those that do not.
As written, that sounds a pretty straightforward thing to do.
But scientifically it isn’t.
Time and money
For a start you need enough feral colonies to produce statistically compelling results. A handful isn’t enough. That might be enough for an anecdote or two and an article in the local beekeeping association newsletter but it won’t convince a peer reviewer or a journal editor.
You need to repeatedly observe the colonies over a protracted period, recording viability and taking relevant samples for molecular analysis (e.g. which pests and pathogens are present?). In addition, being fieldwork, you need to probably do this for more than one season, and ideally you need to look in a variety of different geographic locations.
And to do this you need to pay for the equipment, travel, staff, reagents, food, hotels etc.
Kaching!
Science is expensive, and not because scientists are particularly well paid 🙁 .
If you work on a global human infectious disease there are (multiple) millions of pounds of funding available from government and industry.
If you work on honey bee biology … not so much {{4}}.
Other than the Thompson et al., study mentioned above there are very few studies of feral colonies, and even fewer of what causes the demise of feral colonies or why feral populations are usually not self-sustaining, but are instead dependent upon an annual influx of lost swarms from beelosers.
Until now
Last October I described an interesting study by Kohl et al., (2022) that investigated the longevity of feral colonies occupying known ‘cavity’ trees. These are trees containing black woodpecker {{5}} nesting sites. Several hundred mapped ‘cavity’ trees were observed three times a season – May/June, late September, early April – to determine how many became occupied by swarms, and how many remained occupied the following spring.
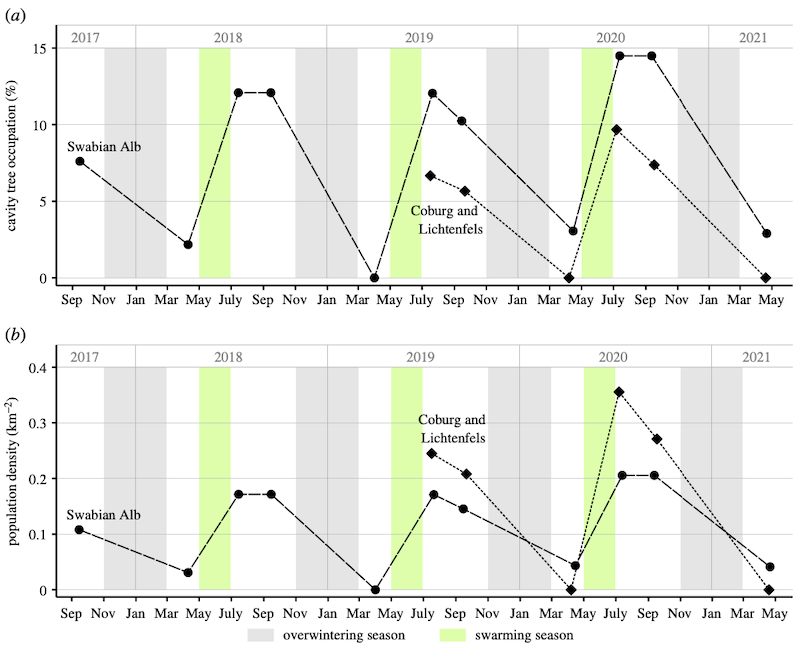
Temporal population fluctuations of feral honey bee colonies in Germany; A) occupancy rates, B) population density
Of the 112 nest sites occupied, 90% survived from May/June until late September, but only 16% were occupied the following spring.
The annual survival rate was ~10%, and the average lifespan of a feral colony was ~32 weeks.
The populations (in three different old growth German forests) were not self-sustaining. The continued presence of honey bees in the forests was dependent upon the annual loss of swarms by beekeepers in the surrounding environment.
Although perhaps disappointing that the populations were not self-sustaining, these bee trees provide an ideal study cohort to determine why the vast majority of lost swarms do not survive to reproduce (swarm).
Kohl et al., 2023
A the follow-up study discussing the potential and actual causes of feral colony loss has just been published (Kohl et al., 2023) with the snappy title:
Parasites, depredators, and limited resources as potential drivers of winter mortality of feral honeybee colonies in German forests
The results are interesting and a little surprising {{6}}. This is because I’ve always assumed that it’s the double-whammy of Varroa and DWV that quickly kills these feral colonies.
It isn’t.
The study is in the main easy to understand and open access, so you can read about all the details I don’t have the time, energy or intellect to explain.
There’s more to be done. Some of the results lack compelling statistical significance, but – like all interesting science – it provides a good basis for further work and clearly points to areas where additional research is needed.
A working hypothesis
Whilst you can just go out into the field and ‘measure stuff’ (tree height and girth, species, cavity entrance orientation etc.), it’s far better to have a working hypothesis of what might account for the high annual losses of recently established feral colonies and so quantify things that are relevant, so allowing these hypotheses to be tested.
The first, and perhaps most obvious, thing that could account for these losses are the range and levels of pathogens known to be detrimental to honey bees.
Secondly, while studying nest site occupancy in the cavity trees the team had previously found beeswax comb on the forest floor under some trees. Is nest site competition (from a range of other species) or robbing responsible for the demise of feral colonies?
Finally, the environment surrounding the cavity tree would be expected to determine forage availability. Previous studies – and careless beekeepers – have shown that a colony needs sufficient stores to get through the winter. A poor environment offering little forage might be associated with higher levels of feral colony loss.
This provides three testable hypotheses:
- Parasites and pathogens limit overwinter colony survival; colonies that perish would be expected to have a wider range or higher levels of honey bee pathogens.
- Nest depredation limits overwinter colony survival; colonies that perish would be expected to be visited/robbed by other species and, conversely, nest sites protected from such visits should survive better.
- Available forage limits overwinter survival; colonies that survive would be expected to occupy landscapes with greater amounts of flower-rich land within foraging range.
Of course, it might be a combination of these, or these and something unknown or unexpected, but this is a good starting point.
Testing the hypotheses
How were these three things tested and what were the results?
I’m going to be reasonably brief here to keep within 23,000 words. I’ll mention the key tests and what I consider the most important or interesting results {{7}}.
The study was conducted between 2017 and 2021. A total of 113 colony winter survival/mortality events were observed, involving 103 unique colonies and 71 cavities. 98% of the cavities were black woodpecker nests in beech trees. Since \~84% of colonies die within a year of occupying a nest site it was inevitable that most testing was conducted on recently established feral colonies – this is particularly relevant when pests/pathogens are considered. There is no distinction made in the paper between feral colonies established for different periods.
Not every feral colony was investigated for pests/pathogens or nest depredation.
Parasites and pathogens
A total of 18 honey bee parasites were quantified – presence and levels – using standard molecular techniques {{8}} from 20 bees sampled in July from each of 67 feral colonies for which overwintering survival was known.
Perhaps surprisingly the range of pathogens present in colonies that died was not higher. Of the 18 species tested, an average of ~5 were present in colonies that subsequently died and those that survived. Not necessarily the same 5, but there was no consistent differences between the community composition in the ‘dead’ or ‘alive’ colonies.
These feral colonies were not riddled with a wide range of pathogens.
Even more surprising – at least initially – of the 13 pathogens detected (remember, not all were present in every colony), the pathogen levels were not higher {{9}} in the colonies that subsequently died.
There’s a large table in the paper you can get all the gory details from.
Varroa was not one of the pathogens tested (you cannot meaningfully sample free-living colonies in tree cavities for mites). I was particularly surprised at the prevalence of DWV (no higher than 20-30%) which, using the most sensitive methods, is effectively ubiquitous in managed colonies when tested.
Although at first glance the absence of significant differences in pathogens present, or their levels, is surprising remember that these samples were taken in July and the majority of sampled colonies will have been present at the site for under one year. Whilst there is a relationship between summer pathogen loads and levels at year-end it is not necessarily linear. For example, colonies that experience a long late season brood break (due to poor forage) may have lower levels than one that was not similarly restricted.
Nest depredation
A small number of occupied nests were fitted with camera traps that recorded winter visitors to the tree/nest.
Of the 15 nests observed, 13 bird and 2 mammal species were recorded with 41% of visits involving the nest cavity being entered {{10}} and so potentially plundered.
Five bird species were observed potentially robbing the honey bee nests; grey-headed, green, great spotted and middle spotted woodpeckers, and great tits. Of these, the latter and green and great spotted woodpeckers are present in the UK.
In addition to these birds, pine martens were observed reaching into, or entering, nests occupied by feral colonies.
There were no real surprises here. These bird and mammal species were already known or suspected of predating honey bee nests. I’m aware some beekeepers have had problems with pine marten, but they’ve never shown any interest in my colonies.
Nest protection
If nest depredation was an issue, preventing entry by the birds or martens should increase colony survival.
They tested this by stapling 8 mm wire mesh over some nest entrances and compared colony survival of protected or unprotected nests. This was done in two successive winters; in the first, survival of protected nests was twice that of those without mesh (33% vs. 15%) but in the second the same percentage survived (10%), meaning that overall there was no difference in colony survival if potential predators were excluded.
This part of the study was limited by the rather small number of nest sites tested (32 with and 40 without mesh) which, coupled with the low overall survival rate, reduced statistical significance. The authors make a number of suggestions on how this part of the study could be improved in the future.
The surrounding landscape
Feral colonies were observed in three study regions in southern Germany (Swabian Alb, Coburg/Lichtenfels and WeilheimSchongau) located 100-300 km apart. In each region, nest sites were up to 50 km apart. The feral colonies occupying the nest sites had access to potentially different forage types within a radius of 2 km (the forest contains relatively little suitable forage, with the bees visiting neighbouring land).
The proportions of land surrounding each nest sites – classified as either deciduous forest, coniferous forest, grassland, cropland or settlement – was quantified.
When surviving and dying colonies were compared, the former were surrounded by an average of ~6% more cropland (i.e. agricultural land on which crops were grown, together presumably with the field margins and hedgerows that separate areas of monoculture). Although this doesn’t sound much, it was significant.
Why so few feral colonies?
The number of feral colonies in an environment depends upon five things:
- Rate at which swarms from managed colonies occupy new sites.
- The survival rate of newly established feral colonies – how many survive their first winter?
- The successful reproduction (swarming) of established feral colonies.
- Survival rate of feral swarms.
- Annual survival of feral colonies after their first winter.
For a population to become self-sustaining it must first get established and must then reproduce at a rate sufficient to make up for the annual losses, or at a faster rate to expand population numbers.
The previous study by these authors – discussed in Feral facts and fallacies – addresses the second (and part of the third) of these points.
This new paper looks at why so few (~16%) survive their first winter.
The range and levels of pathogens in these feral colonies (in July) suggests they do not succumb to disease. Instead the results are suggestive that overwinter robbing (or nest disturbance – a subtle but important difference the authors discuss) is probably detrimental and that the availability of sufficient forage is critical.
I was surprised that pathogens weren’t the major culprit, not least because of the higher levels of DWV reported in the 2014 Thompson et al., study of feral colonies.
However, the age of the colonies in the Thompson paper was unclear. Perhaps they were long-established (or at least not just established) feral colonies?
A useful follow-up study would be to investigate pathogen loads in feral colonies in the first and – for the rare survivors – subsequent seasons. My expectation would be that colony losses attributable to disease would significantly increase from the second season.
The environment and feral colonies
The ‘cropland’ designation used by Kohl and colleagues is a rather generic definition. I don’t know the areas of Germany the study was conducted in but, by extrapolation to UK ‘cropland’, imagine it could cover a very wide range of different habitats.
Compare the difference between hundreds of rolling acres of winter wheat dissected by barbed wire fencing and a patchwork of small fields with wide margins, a scattering of small copses and dense hedgerows.
The latter might well be ‘bee-friendly’, the former probably isn’t.
With the intensification of farming the environment our lost swarms try and occupy is increasingly hostile. I would be interested in the comparative survival rates of feral colonies in the prairie-like expanses of Norfolk and somewhere more closely resembling the bucolic scene in Constable’s ‘Hay Wain’.
The environment also includes the other species competing for nest sites and food – including the food already stored by the bees. We cannot control woodpecker or pine marten numbers (all are protected species), but an expanded study of the survival of mesh-protected feral colonies would show whether this is a significant cause of feral colony demise.
It’s tough out there … even in established self-sustaining feral populations swarm survival rate is low. Fewer than 1 in 4 swarms of Seeley’s bees in the Arnott Forest survived their first winter. Casts – afterswarms headed by virgin queens – fared even worse.
Although pathogen quantification is time consuming and expensive, simply characterising the environment occupied by feral honey bee nests and protecting some of the nests from depredation, would provide important insights into whether – and how – feral populations (can) become self-sustaining.
Note
The authors have another paper – currently available on BioRxiv (i.e. likely submitted but that has yet to be peer reviewed and published) – entitled Reduced parasite burden in feral honeybee colonies. I have yet to read this in detail but may discuss it in a future post.
References
Dainat, B., Evans, J.D., Chen, Y.P., Gauthier, L., and Neumann, P. (2012) Dead or alive: deformed wing virus and Varroa destructor reduce the life span of winter honeybees. Appl Environ Microbiol 78: 981–987.
Kohl, P.L., Rutschmann, B., Sikora, L.G., Wimmer, N., Zahner, V., D’Alvise, P., et al. (2023) Parasites, depredators, and limited resources as potential drivers of winter mortality of feral honeybee colonies in German forests. Oecologia https://doi.org/10.1007/s00442-023-05399-6. Accessed July 12, 2023.
Thompson, C.E., Biesmeijer, J.C., Allnutt, T.R., Pietravalle, S., and Budge, G.E. (2014) Parasite Pressures on Feral Honey Bees (Apis mellifera sp.). PLOS ONE 9: e105164 https://journals.plos.org/plosone/article?id=10.1371/journal.pone.0105164. Accessed October 19, 2022.
{{1}}: And what’s written above is a gross oversimplification.
{{2}}: It usually is.
{{3}}: By the beekeeper, not the bees.
{{4}}: Which is why I spent my career working on the replication, evolution and pathogenesis of human viruses, with sideline projects on the biology of honey bee viruses.
{{5}}: A crow-sized bird that excavates ~10 litre cavities for nesting.
{{6}}: To me at least.
{{7}}: You might have different ideas … read the paper!
{{8}}: qPCR of cDNA generated from extracted RNA … bet you’re glad you read the footnote!
{{9}}: i.e. ‘not statistically different’.
{{10}}: Or partially entered – it depends how big the visitor was and how small the entrance was!
Join the discussion ...